A recent Teacher article explored how teachers can pro-actively plan to address student misconceptions in STEM. In this expert Q&A we dig deeper into the science concepts that primary students struggle with.
Kristy Osborne – physicist, former pre-service teacher and Research Fellow at the Australian Council for Educational Research (ACER) – shares several examples, explains why it’s important to identify and address student misconceptions early on, and highlights how teachers can use them as anchor points for learning progression.
Science, in particular, is a subject area where students can really struggle with some of the concepts – why do you think this is?
One barrier to achieving scientific literacy is the abstract nature of some scientific concepts, such as energy transfer and scale. Visualising objects that are incredibly large (such as some celestial objects) or very small (such as viruses or the particulate nature of matter) can be conceptually challenging for students.
In particular, gases and thermal phenomena are often not well understood in the primary years. Keith Skamp’s work (2005) discusses the issues children have with thinking about the particulate nature of matter, gases in particular. The majority of students think about matter as macrocontinuous – a continuous piece of material, not containing particles or spaces. However, understanding the particulate nature of matter is important for students to develop the conceptual understanding needed to be able to classify materials as solid, liquid or gas.
What does the research say about student misconceptions in science across the world? Do students in different countries struggle with the same things?
Students often develop incorrect ideas about physical science phenomena before they enter the classroom. These alternative conceptions – also known as misconceptions or ‘children’s science’ – are often highly resistant to change and typically pervade students’ views across cultures and countries, indicating that these conceptions are born from everyday experience. For example, some students hold the belief that gases do not have mass, and this belief is held by many students across the world.
Why is it important for teachers to firstly identify student misconceptions, and then plan to address them in lessons?
Ultimately, we want to ensure students leaving school have the scientific literacy needed to fully participate in society. Alternative conceptions can be so difficult to shift that many students carry them into adulthood. That’s why it’s important to identify what misconceptions students might hold in primary school so that they don’t inhibit their learning of science in secondary school. A possible way of addressing these alternative conceptions in the Year 3 classroom is to:
1. Assess whether your class holds an alternative conception.
2. Use a demonstration, analogy or model, to highlight the inconsistency between reality and the tightly held alternative conception.
Predict–observe–explain techniques are an excellent method for achieving both of these points. By asking students to predict what will happen in a system, you are assessing their pre-instructional knowledge and any alternative conceptions they might hold. For example, take the set-up in the figure:
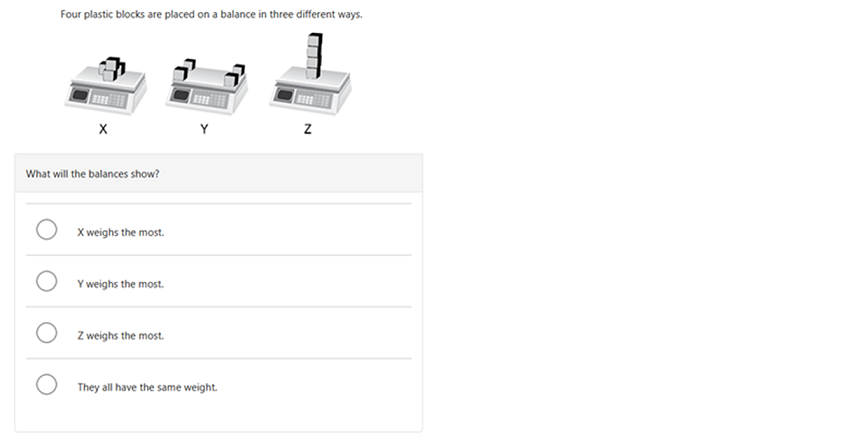
If you take 4 pieces of plasticine, you can place them on the scale and show students how much they weigh, and ask ‘What do you expect will happen to the weight if I squish these 4 pieces together?’ The response will enable you to assess if students hold any alternative conceptions about larger objects always weighing more than smaller objects. When you squish the materials together and place them on the scale, students can observe the measurement. Students then need to explain, with your guidance, why larger objects don’t always weigh more than smaller objects.
Your latest research explores the physical science concepts that year 3 students struggle with. What did the research involve?
In this work, I evaluated the responses of more than 8,000 middle-primary-aged students to a range of physics, space science and chemistry questions. Students were able to choose from answers that included both the key (or accurate conception) and distractors (some of which were common misconceptions). The data were drawn from the International Benchmark Test (IBT), conducted by ACER in 18 countries over 4 testing periods between 2013 and 2018.
In addition to determining the percentage of students that chose the alternative conception, the research also determined the probability of a student answering the question correctly based on their overall performance on the test. This work used unidimensional item-response theory in the form of Rasch modelling, to develop item characteristic curves. These curves allowed for the evaluation of ‘probability of falling for the alternative conception’ versus student ability.
What did you find?
Four alternative conceptions around physical sciences were found for Year 3 students:
- bigger objects always have more mass than smaller objects.
- air/gas has no mass.
- air/gas has no volume.
- day and night are caused by the Earth orbiting the Sun.
Take, for example, the first alternative conception: bigger objects always have more mass than smaller objects. This alternative conception was found through the responses of students to the question shown above, as well as their responses to the following question:
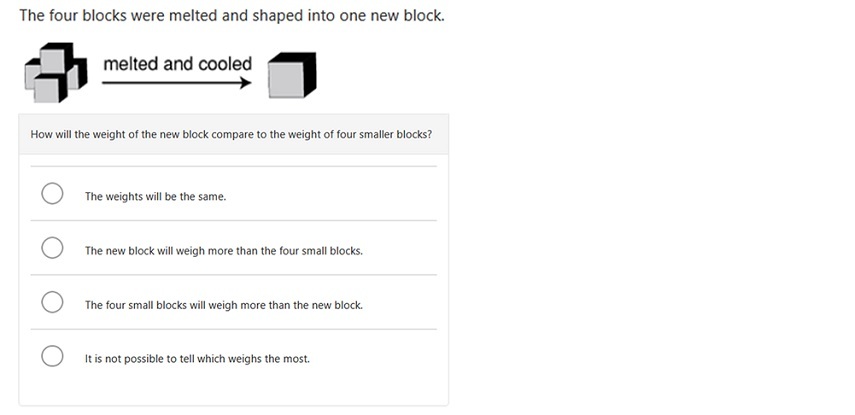
This question was run in tests in 2013, 2014, 2016 and 2018, with between 1,944 and 2,231 students (N) answering the question in each year. The majority of students chose the key (The weights will be the same), however, the alternative conception was the second most popular choice: 25% to 29% of students chose the alternative conception (The new block will weigh more than the 4 small blocks).
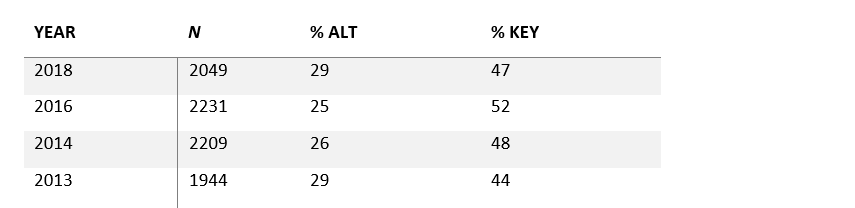
To investigate the ability of students selecting the alternative conception, the probability of a student selecting a particular option versus their ability (latent trait) was plotted for students who attempted the question, and the Rasch model used to fit the data. The Rasch modelling was an excellent fit to the data, indicating that as students’ ability increased, they were more likely to select the key and realise that an object being large does not necessarily mean it weighs the most.
Similar analyses were done for other items on the test, to identify the 4 misconceptions listed earlier.
Do we know if student misconceptions in science have changed over time? For example, did children of the 1990s/2000s struggle with the same concepts?
Students’ misconceptions do not appear to have changed over time. Today’s children still grapple with many of the misconceptions that children of the 1990s grappled with. Take, for example, Ruth Stavy’s 1991 study, which showed that the alternative conception of students not regarding gas as matter – a substance with volume and mass – was held by 76% of year 3 students (Stavy, 1991). In our recent study, 74% of year 3 students also selected the alternative conceptions that air has no mass and/or volume. For the majority of primary-aged students, the definition of matter as being a substance with volume and mass does not hold. Instead, most primary-aged students consider matter to be something they can see and grasp.
Why are misconceptions difficult to shift and how does this impact on future learning in STEM?
These alternative conceptions, also known as misconceptions, are often born from everyday experience. In fact, many alternative conceptions are based on reasoning, making them particularly difficult to shift; if you can’t see gas and grasp it with your hands, it’s harder to understand gas as having a volume and/or mass as compared to a solid object like a piece of wood.
Your research identified anchor points for learning progression. What are they and why is this useful? And how else can teachers support students in this area?
Anchor points are concepts that students need to grasp so they can progress in their learning. Air and other gases having mass could form an anchor point in a learning progression and, for students to progress in the core concept of gravity and/or states of matter, it will be important for them to overcome this alternative conception. Similarly, misconceptions around larger objects not necessarily weighing more than smaller objects, could form an important anchor point in a learning progression around density.
Ultimately, this research has shown that many of the physical science alternative conceptions held 30 years ago are still prevalent in the modern classroom. A concerted effort is still required to change students’ thinking on these concepts and ensure students’ leaving school have the scientific literacy needed to fully participate in society. I hope teachers will be able to use this research to help them plan to address these misconceptions, using them as anchor points in a learning progression.
References
Skamp, K. (2005). Teaching about stuff. Primary Science Review, 89, 20–22.
Stavy, R. (1991). Children’s ideas about matter. School Science and Mathematics, 91(6), 240–244.